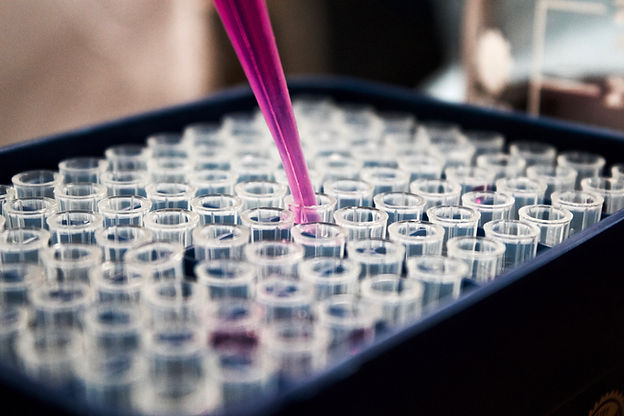
Cargobay Version 1
Our first attempt at creating a switchable-configuration DNA nanostructure able to capture target molecules from solution

DNA Origami
The heart of our innovation lies in the art and science of DNA origami. The same molecule that so amazingly encodes the instructions of life can be pre-programmed to fold into desired shapes like our cargobay!
To achieve this we add short length DNA segments known as staples, which are specially designed to bind at certain points to our much longer scaffold DNA.
These staple strands act as molecular fasteners, which guide the main DNA strand into the desired shape.
This is achieved by simple Watson-Crick base pairing between sections of the staple DNA and the scaffold DNA.
Tether Design
​
Our DNA nanostructure features a strand of DNA approximately 80 nanometers in length, held inside the width of the cargobay.
This tether features several aptamers (shown in grey), short sequences of DNA that conform into a shape especially sticky to nanoplastics in the blood.
​
This feature enhances the ability of the cargobay to capture plastic particles from the surrounding solution
36 different designs
​
To enhance the versatility of our container and test its functionality when varying different parameters, we can modify it by adding different types of staples to the supporting structure. Our primary barrel design comes in three configurations: 'locked open,' 'sealed closed,' and 'loose,' which is an intermediary state between the other two. In this 'loose' state, certain parts of the DNA structure can interact in a way that favors the closed configuration due to a chemical behavior of DNA called pi-pi stacking. To address this, we're experimenting with the addition of polyT (TTTTT) tails at specific locations on the DNA strands. We anticipate that these tails will introduce steric crowding, decreasing the enthalpy of this interaction, and favoring the more entropic loose state.
Furthermore, we're exploring how adjusting the tightness of the flexible extension (tether) impacts our device's ability to capture substances from the surrounding solution. This variation in tether tightness is a critical design consideration that we're meticulously testing during the folding process of our device.
01
It's Bigger, Hopefully it's Better
In order to take full advantage of the length of scaffold available, version 2 is three times longer than version 1, with more aptamers on the inside and less free scaffold influencing the structure we expect better performance and yield.
02
Same Design Principles
By retaining the major design principles but fine tuning the structure
we have retained our experience and understanding from the first prototype design.
03
Promising Preliminary Results
Preliminary gel electrophoresis results of the Cargobay Version 2 demonstrate expected outcomes. The reduction of free tether has allowed differences between conformational states to be more clearly observed, agreeing with our hypothesis.
Cadnano
For the design of our Cargo Bay structure, we used the Cadnano design software. It provides a simplified interface that allows for the creation and visualisation of DNA origami structures. In order to do so, the DNA gets wound up, and so we need to make sure that we match the crossovers to where the DNA helices are. Within Cadnano’s architecture, it actually already accounts for this natural twisting of the DNA helix of about 10.5 base pairs, and, from that, determines when crossover points could be. Cadnano is split into two views: the Slice Panels and the Paths Panel. Each of these allow the following inputs:
01
Slice Panel:
-
Honeycomb or Square Lattice
-
How many cylinders, or DNA helices, are wanted
02
Paths Panel:
-
Base pairs represented by each square, with bolded lines representing 7 base pairs
-
Scaffold design
-
Crossovers between the scaffold
-
Staple design
-
Crossovers between the staples

Adding Scaffold:​
In order to start the designing process, a cross-section must be developed within the Slice Panel. This will guide the 3D structure of the design. In order to add scaffold onto the Paths Panel in the location where the rectangle line is, one must click again on the yellowed cylinders in the Slice Panel. The numbering in the Slice Panel corresponds to those added in the Paths Panel, so the scaffold will appear depending on which cylinder number was selected. This scaffold can be extended by selecting and dragging the square or triangle of the scaffold. The triangle represents the 3’ end and the square represents the 5’ end of DNA.
​
Scaffold Crossovers:
Cadnano shows possible crossover locations through the light blue edges on the Cadnano design, and the scaffold can be connected automatically by clicking on them. Otherwise, using the pencil tool, one can individually choose to connect the scaffold by clicking on a triangle and then connecting this to a square. Crossovers can be broken by using the break button.
​
Adding Staples:​
Staples can be added according to underlying algorithms within cadnano by pressing the auto staple button. Otherwise, using the pencil tool, staples can be added by dragging above the scaffold. Staple length is limited to being between 60 and 90 bases, as otherwise, they are unfavourable energetically. If they are not in the required range, they will appear highlighted and boldened.
​
Staple Crossovers:
Using the pencil tool, one can individually choose to connect the staples by clicking on a triangle and then connecting this to a square. Crossovers can be broken by using the break button.
​
Crossover Density:
For the scaffold and staples, we must consider the crossover density over the structure’s area, as too many crossovers lying within too small an area may lead to atypical formation of the barrel due to instability in terms of electronegativity during folding.
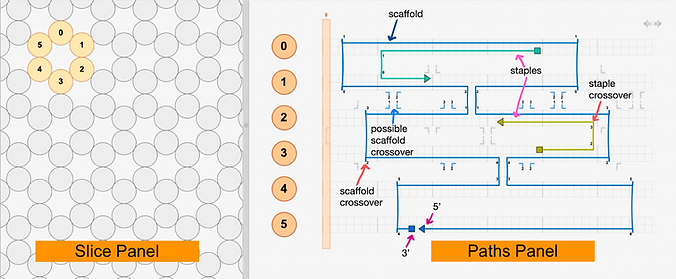
Precursor Design
The Cargo Bay design was based on a precursor DNA origami design (developed by our lab member Matt Turner), the Cadnano design.
This is the open version:
​
​
​
​
​
​
​
​
​
​
This is the closed version:
​
​
​
​
​


Cargo Bay Version 1
​
We made multiple modifications to develop our Cargo Bay design using the phage m13 p7308 scaffold. One modification was to change the location of the excess scaffold from inside the barrel to being at the top left corner. Also, we added three different tether designs to the centre of the barrel: flexible, shortened and tightly bound. To these, we added 17 specific handles here as well as inside the barrel so as to be able to attach aptamers here.
Here is an outline:
​
​
​
​
​
​
​
​
​
For the flexible tether, we added we initially were not planning to add any staples, however, based on our results from NUPACK (as described below), we had to add staples in between the handles in order for the scaffold to be double-stranded so as to not be able to self-hybridise, as shown below.
​
​
​
​
​
​
​
​
​
​
For the tightly bound tether, we added staples to keep the tether bound together tightly in between the handles, as shown below.
​
​
​
​
​
​
​
​
​
​
​
For the shortened tether, we added a mixture of the staples used in the flexible and tightly bound tethers experimentally, and therefore had no specific Cadnano design.
​
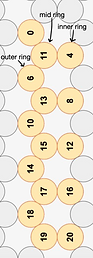
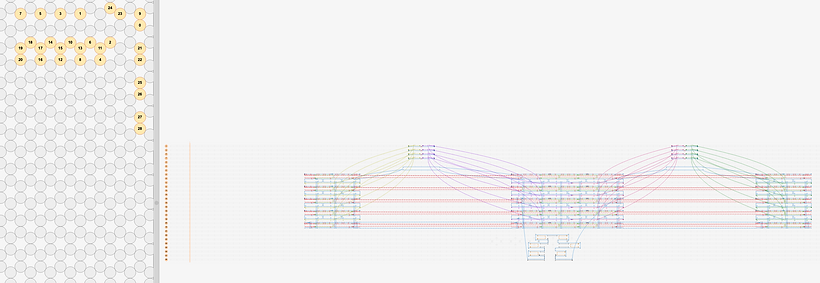
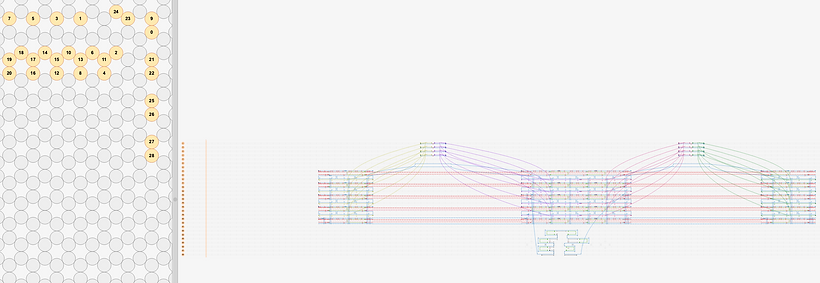
Cargo Bay Version 2
In order to create this second version of our Cargo Bay design, we used the phage m13 p8634 scaffold to be able to extend our initial design by a factor of 3.5. In order to do so, we repeated the Cadnano design downwards by following the structure for each grouped inner, mid and outer ring. While the tether designs remained consistent with the first version of our Cargo Bay design, we needed to adjust due to our using of another scaffold, meaning that the staple and handle sequences changed.
Here is the design in Cadnano:​
​
​
​
​
​
​
​
​
​
​
​
​
​
​
​
​
​
​
NUPACK
For the design of our Cargo Bay structure, we used NUPACK (or Nucleic Acid Package), which uses an algorithm to predict how single-stranded DNA or RNA interact, to determine whether the single-stranded DNA in our design would remain single stranded or self-hybridise by forming hairpin structures.
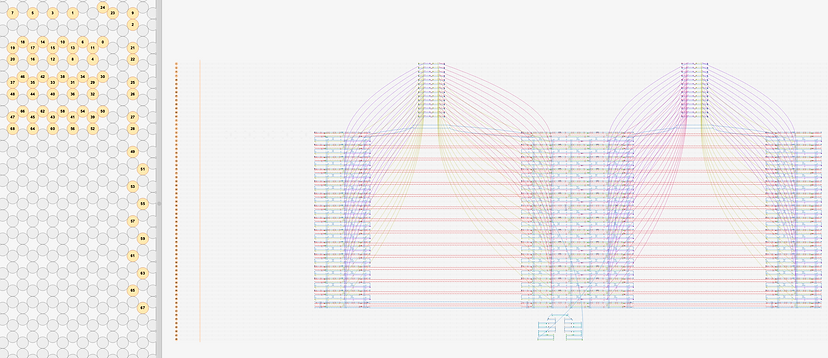
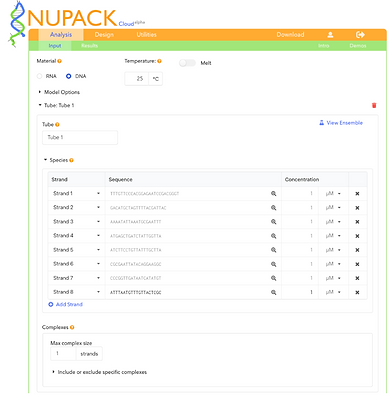
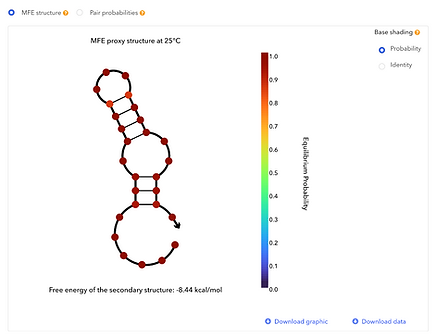
Our DNA Cargo Bay design features a tether with attached handles for binding to substrates. The tether exists in the following three different conformations:
​
-
Flexible with mostly ssDNA and regularly spaced handles
-
Shortened with some stapled sections to increase tension and reduce flexibility, with regularly interspaced staples and some ssDNA sections
-
Tightly Bound with the length entirely stapled into a fixed configuration and the same regularly interspaced staples
In order to test our design, we checked some of the following sequences of single-stranded DNA from our scaffold in NUPACK. The output we got from Cadnano contained the complementary strands of the scaffold, so in order to get the sequence of the scaffold itself, we found the reverse complementary strands. Then, we put these sequences into NUPACK to determine if they would self-hybridise or remain single-stranded, as shown below:
​
​
​
​
​
​
​
​
​
​
​
​
Due to the formation of hairpin structures on multiple of the single-stranded DNA sequences, we decided to keep those sections of the tether as double-stranded DNA through the addition of staples with single base pair gaps for flexibility.