
Relevance
Nanoplastics are found all around us - from “every ecosystem on the planet from the Antarctic tundra to tropical coral reefs.” (1) Humans ingest plastics every day through water, food, the air we breathe and even through the skin barrier. (2) These plastics were just recently detected in human blood as well as in organs, highlighting how ingested plastic particles can travel through the entire body and can accumulate in different organs. (3) In the body, these nanoplastics are linked to, among others, inflammation, oxidative stress, and neurotoxicity in human cells. (4)
Currently, there are no products that detect and capture plastics present in the bloodstream of humans. Many mechanisms to tackle the problem of plastic pollution address the reduction of plastics from the environment, such as from water. While this would help reduce the amount of nanoplastics taken in by humans and other organisms in the future, it does not address the plastics already present within humans.
Therefore, in this project, we aim to use DNA origami to design a novel DNA structure - the DNA cargo bay - that is able to detect and capture plastics in the bloodstream through the use of DNA aptamers.
DNA origami describes the technique of “folding a long single strand of DNA (scaffold) into precise shapes.” (5) The formation of these shapes is facilitated through the use of staples - shorter single-stranded DNA - which fold the scaffold structure and hold it in place through the use of crossovers. (6) In this project, we designed the DNA cargo bay structure in this way using the cadnano design software. (7)
DNA aptamers are “short synthetic single-stranded oligonucleotides that specifically bind to various molecular targets” with “high affinity and specificity.” (8,9) As DNA aptamers are “neither immunogenic nor toxic,” they can be useful in a wide range of fields, including in “pathogen recognition [and] monitoring environmental contamination”. (9, 10) Additionally, aptasensors have been shown to be able to detect plastics: for instance, BPA, a chemical typically known for its use “in the production of polycarbonate plastics,” was detected in “milk, orange juice and mineralized water samples” with few false negative and few false positive results. (11, 12) Therefore, since the detection of plastics using aptamers is possible, we decided that our DNA cargo bay design would use DNA aptamers for the detection and capture of nanoplastics.
​
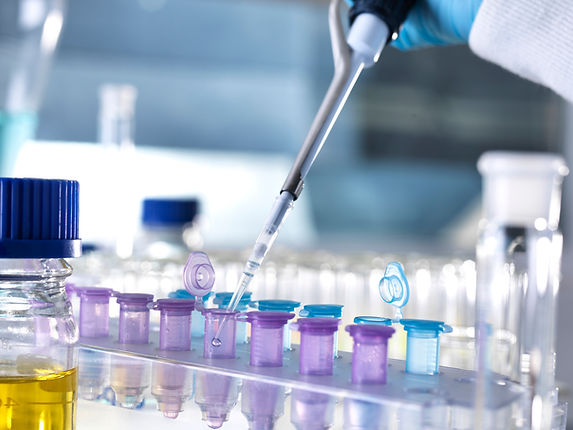
Merit
We first need to tackle the possibility of capturing molecular cargo inside our body and thus, we propose a switchable nano-barrel structure to patrol the bloodstream. This would enable its capability of opening to selectively capture plastic particles and then sequentially close to isolate them from the bloodstream.
​
In conjunction with having an idea of the capture concept we wanted to go with, USYD DNA Nano group’s exploration into the realm of DNA origami barrels intrigued us greatly and from that point onwards began the hunt for a barrel design capable of meeting our design requirements. We had initially considered a one-hinge system either in the form of a lid attached to an enclosed barrel or a hinge down the axis of the barrel to open completely to two semi-circles. However, we decided that even when the lid was open, particles may struggle to get inside the barrel but a barrel that completely opened may not hold onto captured particles as well. It was not long after this that the idea of mimicking NASA’s space shuttle was thrown around and well that was history.
​
It couldn’t be a better fit, with the cargo bay being cylindrical in nature, can open and close whilst maintaining a space for the goods to reside in addition to even a robotic arm used to capture payloads.
​
Our project and laboratory work specifically explores the capacity to capture and enclose particles to validate that our system is capable of selectively removing targets from a solution. Ultimately, we envision to further modify our barrel to switch to a closed conformation upon binding of a certain concentration of nanoplastics that can then be cleared by the body.
This is an important solution because it paves the way to potentially capture specific unwanted particles from the body that inevitably find their way in with the state of our plastic-centric world. It offers substantial prospects due to the nature of DNA origami that provides us with the capacity to easily modify structures with the addition of aptamers to exclusively aim for target molecules.
​
Furthermore, common applications of nanotherapeutics include drug delivery and radiologic imaging yet the aspect of capturing appears to be comparatively less explored.
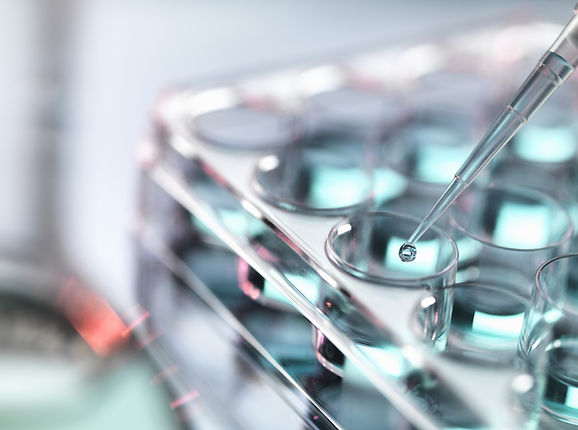
Future Work
The immediate next steps would be to image our extended Cargo Bay Version 2 design under TEM. From this, we may be able to determine whether the folding results are as expected for the three different states. In addition, we want to be able to confirm the binding of gold particles to our design, so as to be able to successfully demonstrate target molecule binding.
In diagnostics, one future application of the DNA Cargo Bay’s detection mechanism may be to calculate the concentration of particles in the blood. Additionally, this Cargo Bay design may be applied to a broader setting within research, as there is a large choice of aptamers to pick from.
The ultimate goal would be to be able to remove nanoplastics from the bloodstream and body, and we imagine it could possibly work through receptor mediated clearance by the liver or through the use of liposomes. A potential method to ensure that the Cargo Bay isn’t removed from the body too quickly, without allowing for suffient plastic capture, could be by linking the concentration of plastics captured to a tag that is only revealed when a specific concentration is reached within the Cargo Bay. For instance, once a specific capture load is reached, the design could switch from the open to the closed state, thereby exposing such tags on the outside of the Cargo Bay that allow for receptor mediated clearance by the liver or removal through liposomes. Otherwise, possibly plastic neutralising enzymes could be introduced that are able to degrade plastic within the Cargo Bay design, so that the DNA origami structure could then be reused to detect and capture other particles within the bloodstream.
References:
-
US EPA, ORD. Microplastics Research. 22 Apr. 2022, https://www.epa.gov/water-research/microplastics-research.
-
Dietary and Inhalation Exposure to Nano- and Microplastic Particles and Potential Implications for Human Health. https://www.who.int/publications-detail-redirect/9789240054608. Accessed 28 Oct. 2023.
-
Leslie, Heather A., et al. ‘Discovery and Quantification of Plastic Particle Pollution in Human Blood’. Environment International, vol. 163, May 2022, p. 107199. ScienceDirect, https://doi.org/10.1016/j.envint.2022.107199.
-
Lee, Yongjin, et al. ‘Health Effects of Microplastic Exposures: Current Issues and Perspectives in South Korea’. Yonsei Medical Journal, vol. 64, no. 5, May 2023, pp. 301–08. PubMed Central, https://doi.org/10.3349/ymj.2023.0048.
-
Mekhail, Mina, et al. ‘Chapter 25 - Self-Assembled Nanostructures (SANs)’. Biology and Engineering of Stem Cell Niches, edited by Ajaykumar Vishwakarma and Jeffrey M. Karp, Academic Press, 2017, pp. 391–409. ScienceDirect, https://doi.org/10.1016/B978-0-12-802734-9.00025-1.
-
Tadakuma, Hisashi, et al. ‘Chapter Five - RNA Study Using DNA Nanotechnology’. Progress in Molecular Biology and Translational Science, edited by Satoko Yoshizawa, vol. 139, Academic Press, 2016, pp. 121–63. ScienceDirect, https://doi.org/10.1016/bs.pmbts.2015.11.004.
-
‘Cadnano’. Cadnano, http://cadnano.org/welcome. Accessed 28 Oct. 2023.
-
Ni, Xiaohua, et al. ‘Nucleic Acid Aptamers: Clinical Applications and Promising New Horizons’. Current Medicinal Chemistry, vol. 18, no. 27, 2011, pp. 4206–14. PubMed Central, https://www.ncbi.nlm.nih.gov/pmc/articles/PMC3260938/.
-
Lakhin, A. V., et al. ‘Aptamers: Problems, Solutions and Prospects’. Acta Naturae, vol. 5, no. 4, 2013, pp. 34–43. PubMed Central, https://www.ncbi.nlm.nih.gov/pmc/articles/PMC3890987/.
-
Zhang, Yang, et al. ‘Recent Advances in Aptamer Discovery and Applications’. Molecules, vol. 24, no. 5, Mar. 2019, p. 941. PubMed Central, https://doi.org/10.3390/molecules24050941.
-
‘Bisphenol A (BPA)’. National Institute of Environmental Health Sciences, https://www.niehs.nih.gov/health/topics/agents/sya-bpa/index.cfm. Accessed 28 Oct. 2023.
-
Jia, Min, et al. ‘High Affinity Truncated Aptamers for Ultra-Sensitive Colorimetric Detection of Bisphenol A with Label-Free Aptasensor’. Food Chemistry, vol. 317, July 2020, p. 126459. ScienceDirect, https://doi.org/10.1016/j.foodchem.2020.126459.